Voltera | Printing a Magnesium Zinc Battery with Saral Inks on PET
- khashayar Ghaffarzadeh
- Nov 25, 2024
- 6 min read
Author: Jesus Zozaya, CEO at Voltera | sales@voltera.io
As presented at the TechBlick Berlin event in October, our project, a multilayer magnesium zinc battery, validated the compatibility of Saralon’s battery inks printed on a PET substrate.
Watch our Video: Printing a Flexible Magnesium Zinc Battery with Voltera NOVA
MATERIALS USED
SUBSTRATES USED
TOOLS AND ACCESSORIES
Project Overview
Purpose
The purpose of this project is to demonstrate how we fabricated a functional multilayer printed battery on a flexible PET substrate, validating the compatibility of PET with a variety of conductive and insulating inks.
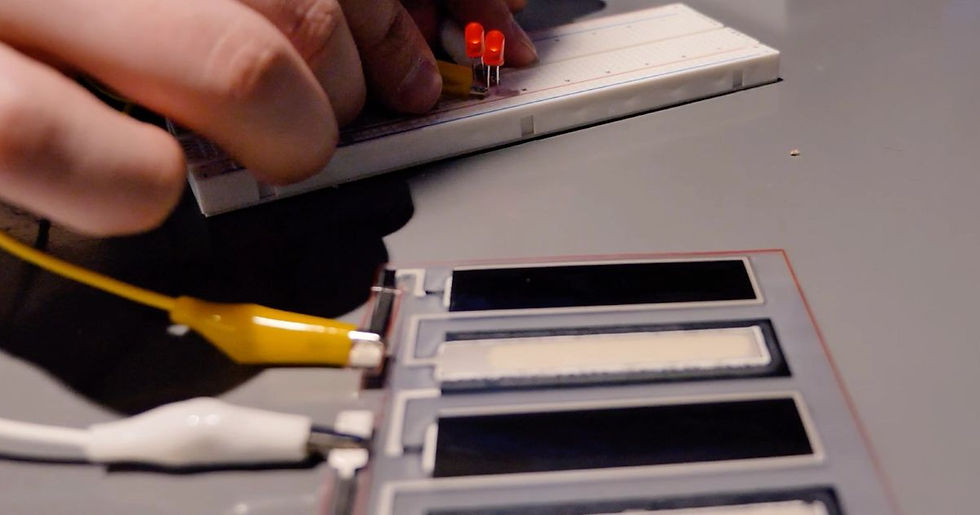
Image: Printed flexible battery-powering lights in a breadboard
Design layout
Typically, a printed battery consists of five layers:
Current collector layer for the anode
Anode layer
Separator layer with electrolyte
Current collector layer for the cathode
Cathode layer

We segmented the battery into halves, each comprising four units, connected in series. As a result, our final print consisted of seven layers.
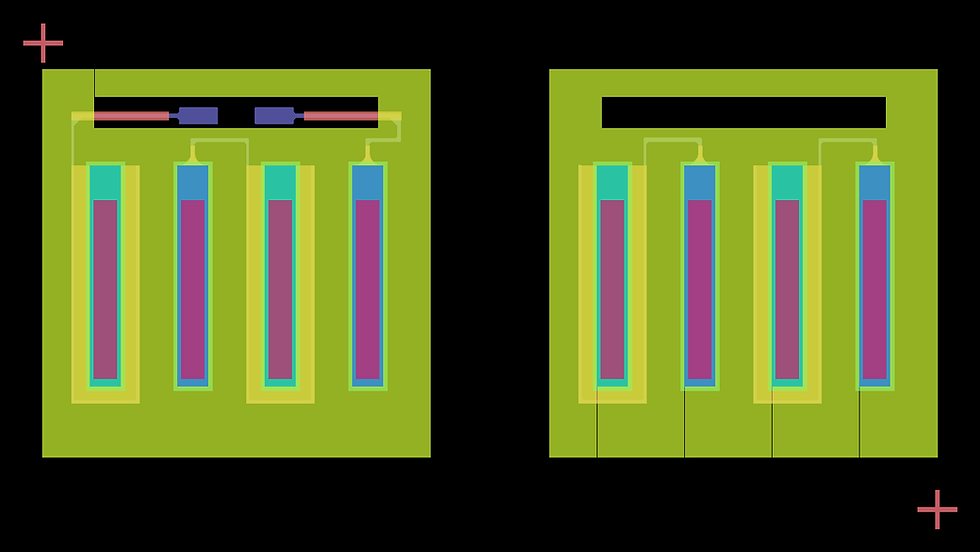
Image: Design layout
The printing sequence was as follows:
Silver conductive layer
Carbon current collector layer
Zinc anode layer
Manganese dioxide cathode layer
Separator layer
Hot melt glue layer
Zinc chloride electrolyte layer
Desired outcome
Once the halves are laminated together, the cells align perfectly and do not exhibit any leakage. When connecting its two probes to the battery terminals, the multimeter shows a stable reading of about 2.4 V.
Functionality
The device incorporating the Saral inks was able to operate at a voltage of 2.4 V, which can make the battery quite versatile. With a voltage of 2.4 V, a printed battery can power a range of devices from low-power electronics such as LEDs, to more demanding applications such as handheld GPS devices, depending on its capacity.
The dimensions for the battery are 90 mm L × 85 mm W × 0.75 mm H, with an initial capacity of about 1 mAh. Larger energy capacity and different voltages are achievable by varying the size and thickness of the layers, choosing different materials for electrochemical reactions, or adding more cells in series.
We are speaking at the Free-To-Attend
Printing the magnesium zinc battery
Silver conductive layer
This layer consists of a silver conductive circuit connecting the positive and negative terminals. The Saral Silver 600A Battery Ink used for this layer provides a moderate electrical conductivity (< 20 mΩ/sq at 25 μm), ensuring reliable current flow between the electrodes.
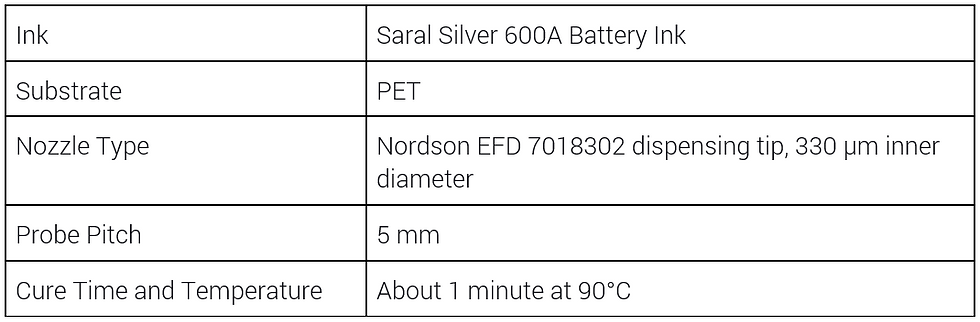
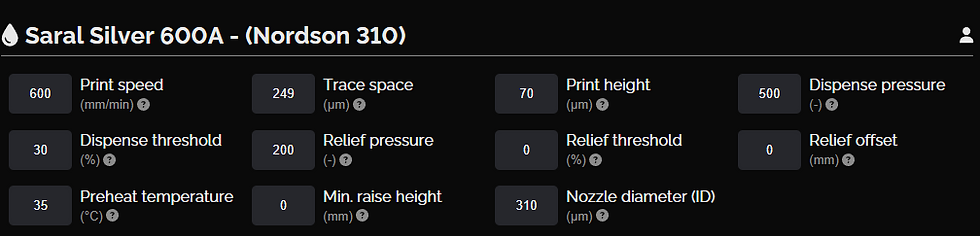
NOVA settings, silver conductive layer
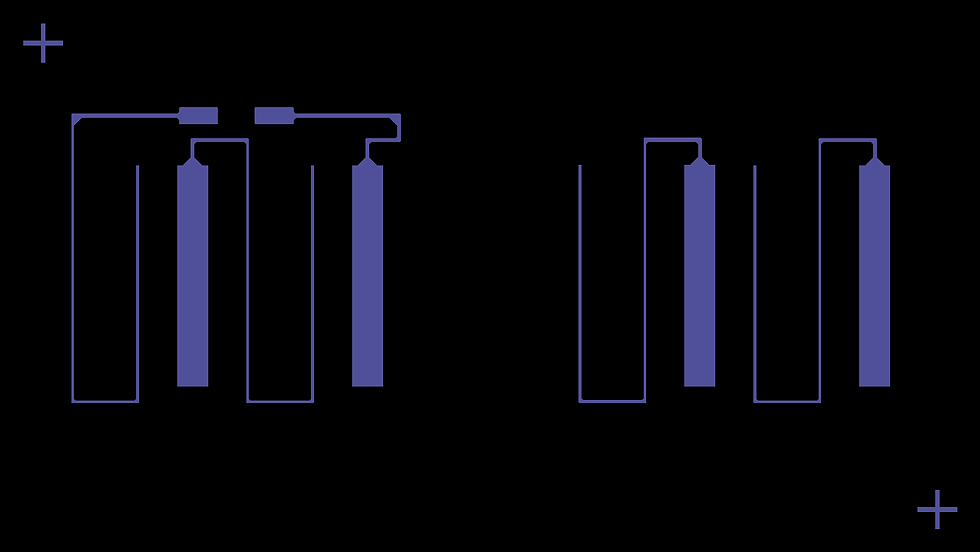
Image: Silver conductive layer design

Image: Silver conductive layer print result
Carbon current collector layer
This layer consists of carbon features that collect current from both the anode and cathode, covering all eight cells (the basic units that generate electrical energy through electrochemical reactions) across the halves. The Saral Carbon 700A Carbon Based Conductive Ink provides excellent adhesion on PET, with a moderate resistance of 30 Ω/sq at 25 μm, ensuring efficient collection of current.
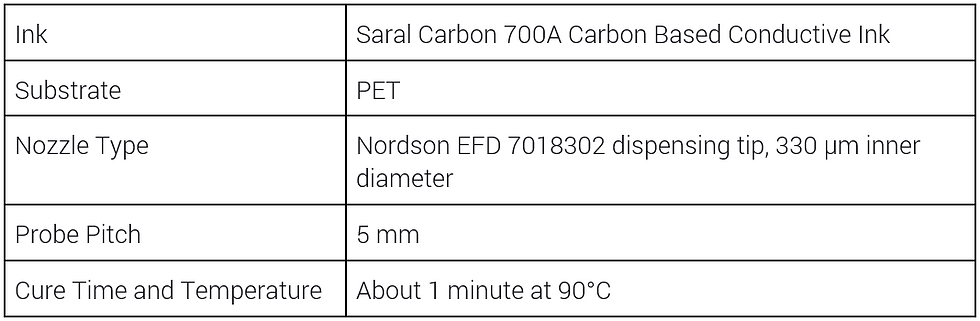

NOVA settings, carbon current collector layer

Image: Carbon current collector layer design
Zinc anode layer
This layer includes four cells that serve as the anode of the battery. The Saral Zinc 700 Battery Ink provides a sufficiently thick layer by one print, ensuring that the anode maintains a high conductivity and stability throughout its lifecycle. The high zinc content promotes efficient electron flow, which is critical for maintaining the battery's overall energy output and longevity.
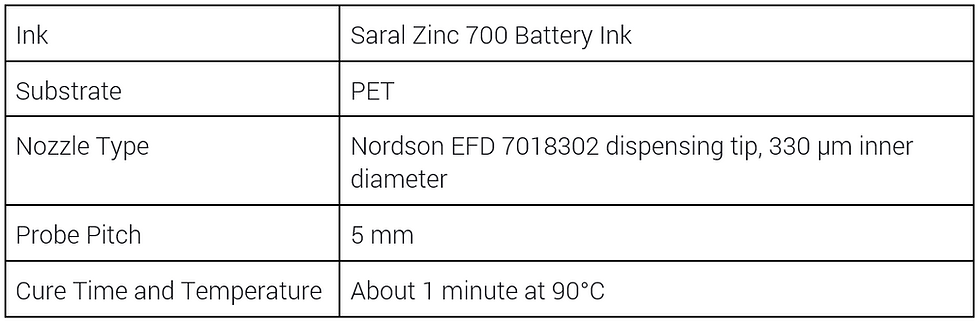
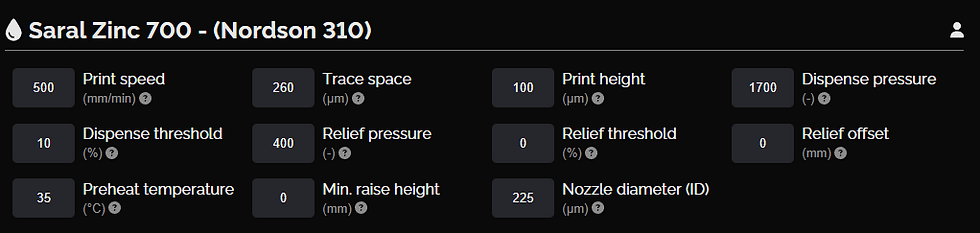
NOVA settings, zinc anode layer

Image: Zinc anode layer design

Image: Zinc anode layer print result
Manganese dioxide cathode layer
This layer includes four units that serve as the cathode of the battery. Similar to the The Saral Zinc 700 Battery Ink, the Saral MnO2 800 Battery Ink provides a sufficiently thick layer by one print, ensuring that the cathode maintains a high conductivity and stability throughout its lifecycle.


NOVA settings, manganese dioxide cathode layer
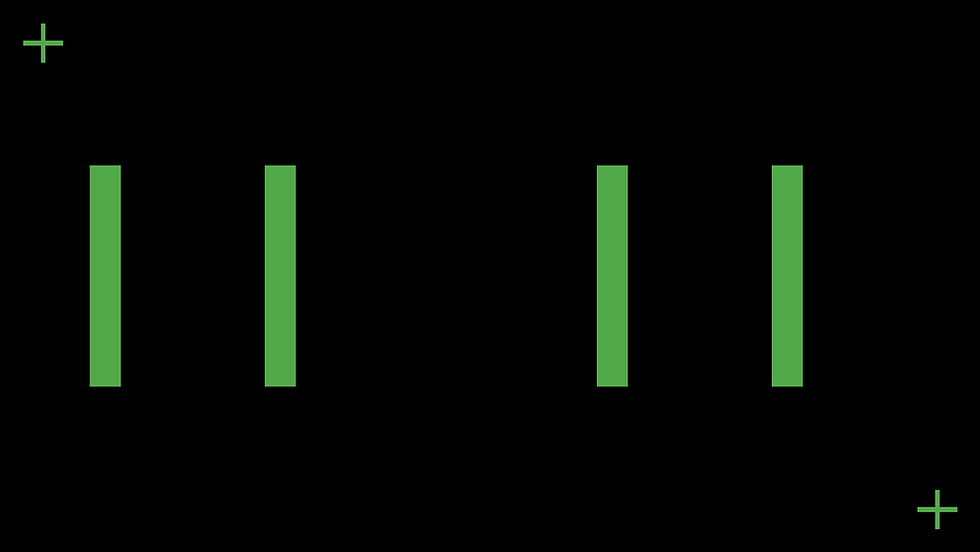
Image: Manganese dioxide cathode layer design
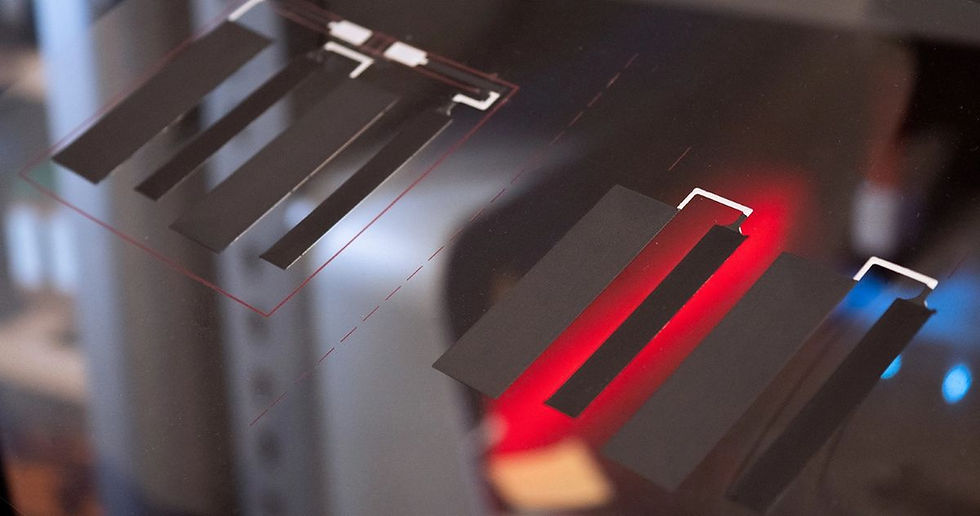
Image: Manganese dioxide cathode layer print result
Separator layer
This layer consists of eight units printed with a water-based ink to create separation between the anode and the cathode layers. Once dried, it becomes porous to allow ion travel.

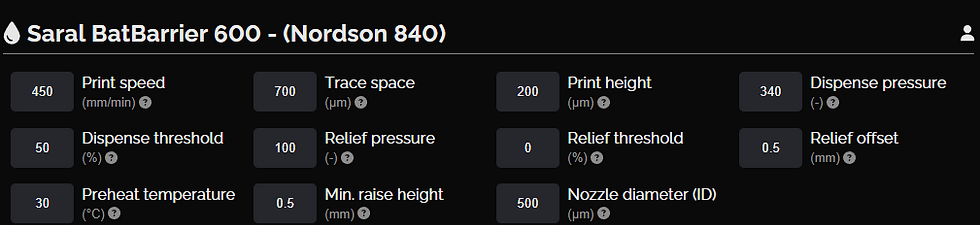
NOVA settings, separator layer
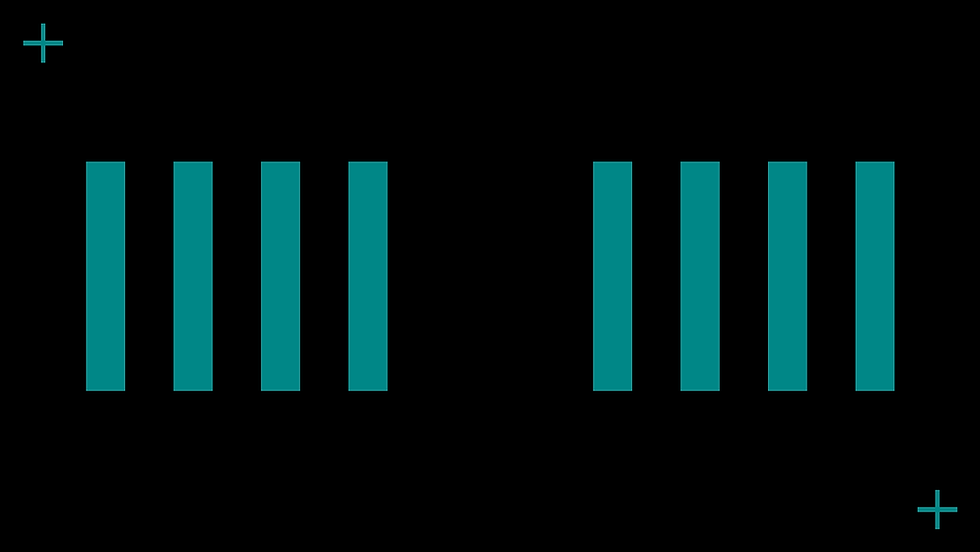
Image: Separator layer design
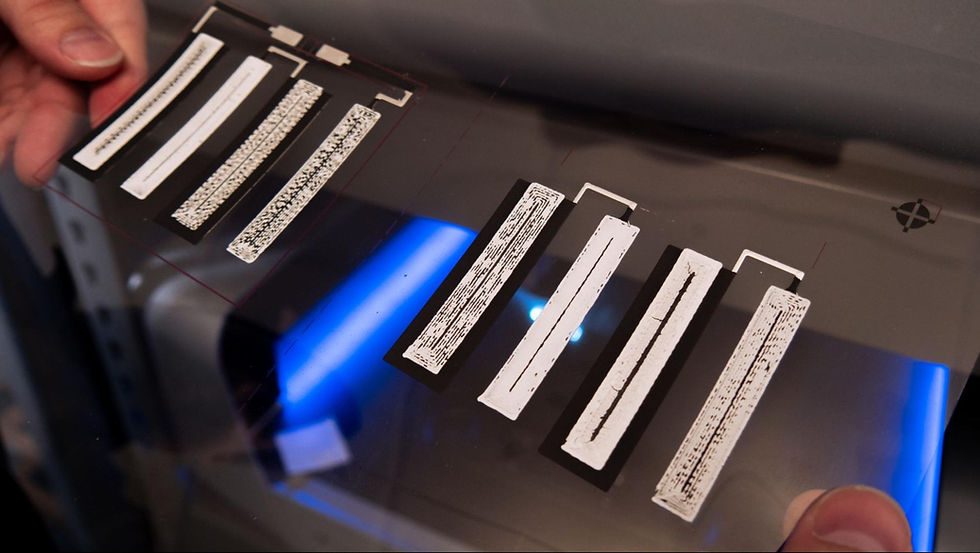
Image: Separator layer print result
Hot melt glue layer
Excluding the terminals, anodes, and cathodes, this layer covers the whole surface of the final print and facilitates lamination.
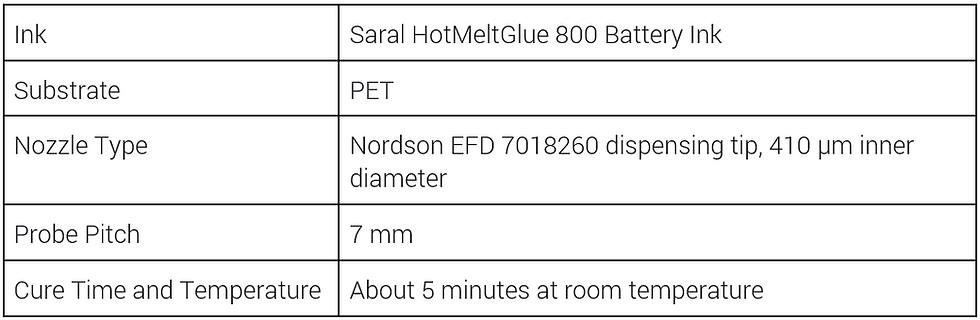

NOVA settings, hot melt glue layer
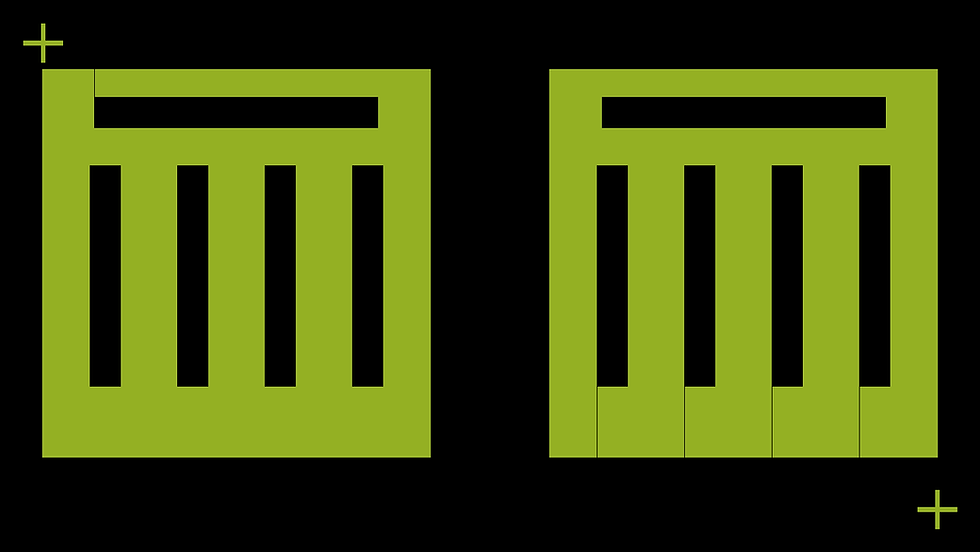
Image: Hot melt glue layer design
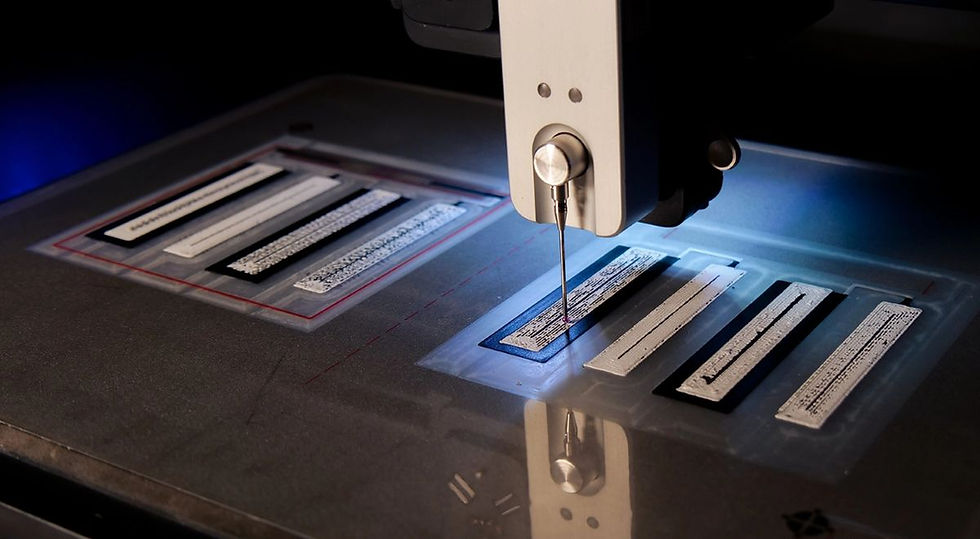
Image: Hot melt glue layer print result
Zinc chloride electrolyte layer
This layer consists of eight units of electrolytes, each slightly smaller than the anode/cathode units. They provide electrolytes that facilitate the movement of ions between the anode and cathode.

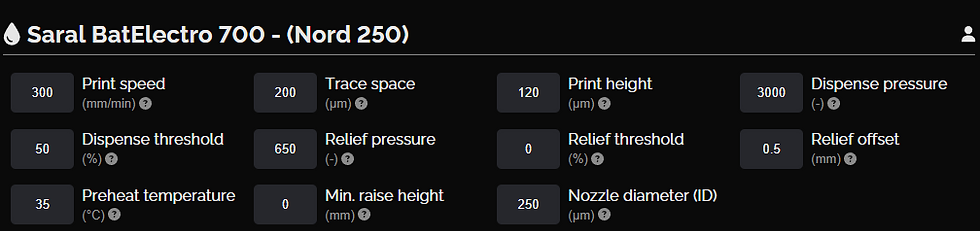
NOVA settings, zinc chloride electrolyte layer

Image: Zinc chloride electrolyte layer design
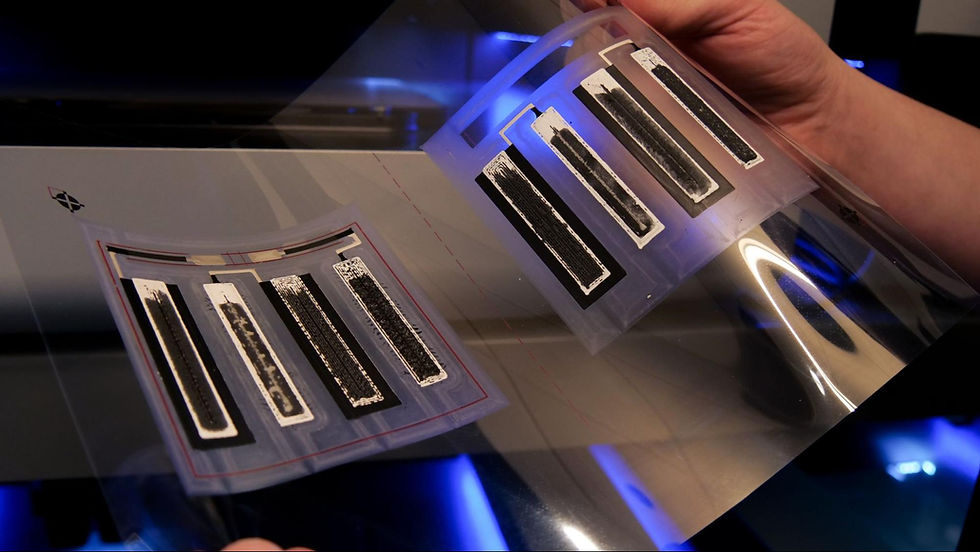
Image: Zinc chloride electrolyte layer print result
Laminating the halves of the printed battery together
Once the last layer was printed and dried, we cut out part of the PET substrate to leave the terminals exposed. We then folded the print from the middle, carefully aligning the fiducial marks.
Next, we inserted the PET into the laminator at 120°C, with the terminals going in last. This was to prevent leakage of the electrolyte to neighboring units and causing short circuits in the battery. When subjected to heat, the hot melt glue layer becomes sticky and holds the halves together.
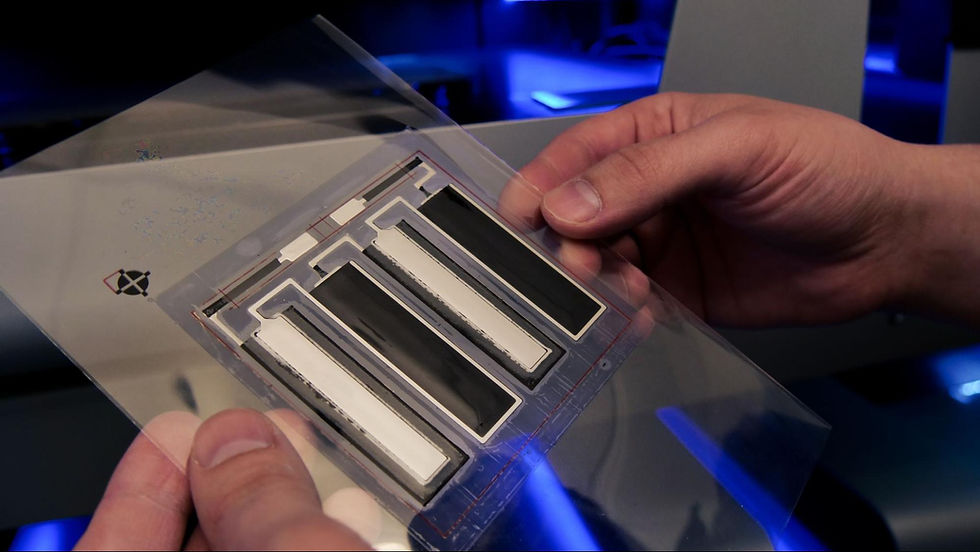
Image: Printed flexible battery folded and laminated
Challenges and advice for printing batteries on flexible substrates
Nozzle compatibility
Nozzle clogging was an issue while working on the separator layer, which was printed with an ink that tends to dry quickly and is prone to forming agglomerates if left unused for an extended period.
In addition, due to the thin, water-like consistency of the water-based BatBarrier 600 Battery Ink, we had to refine print settings to adjust for over-dispensing in order to achieve the intended thin, uniform traces.
We mixed the ink using a dual asymmetric centrifugal mixer before printing. This process, which lasted for 10 minutes at a speed of 2,000–2,500 rpm, proved effective in breaking up the agglomerates and alleviating clogging. Additionally, removing excess water from the ink (we removed 1.8 mL) helped resolve issues with over-dispensing.
Layer leakage
Because the electrolyte layer was printed with a water-based zinc chloride ink, it posed some challenges during lamination. To avoid leakage, we noted that sufficient time was required for the electrolyte layer to absorb into the separator layer before laminating the halves together.
As the design varies, the volume of inks dispensed is different. It is therefore difficult to pinpoint an exact time required for the electrolyte layer to absorb. Some visual signs that indicate full absorption include:
● A noticeable lowering of the level of the electrolyte layer
● Drying and uniformity of the print surface
● A change in the color of the electrolyte layer to a dull white hue
It is also important to note that achieving a higher short circuit current usually involves increasing the thickness and size of the electrolyte layer. Consequently, more time should be allowed for the layer to dry and settle properly.
Conclusion
The thickness of the final print (excluding the PET substrate) is 500 µm, which highlights the potential of printed batteries to be ultra thin and flexible. More importantly, this flexibility renders printed batteries highly customizable — they can store energy in various shapes and sizes to suit product design, instead of the other way around.
In this project, we experimented with zinc and magnesium dioxide inks as the anode and cathode, but a wide variety of materials can be used for printed batteries, including lithium cobalt oxide, carbon nanotubes, and vanadium oxide, to name a few. As we continue to explore the possibilities of printed batteries, we invite you to view the other application projects we’ve completed.
As we continue to explore the possibilities of printed batteries, we invite you to view the other projects we’ve completed.
If you’d like to discuss your designs or our NOVA materials dispensing system, please book a meeting with our applications team or contact us at sales@voltera.io.
We are exhibiting!
Visit our booth at two flagship TechBlick's events
(1) in Boston on 11-12 June 2025 and (2) in Berlin on 22-23 October 2025.
Let's RESHAPE the Future of Electronics together, making it Additive, Sustainable, Flexible, Hybrid, Wearable, Structural, and 3D.
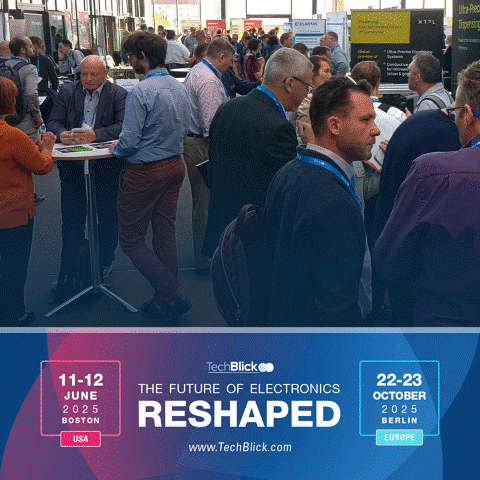
Comments