Please scroll down to see the agenda.
This will TechBlick’s fourth online event covering four major themes in the battery industry:
(1) Non-Li chemistries inculding Na, Al, etc
(2) Next-gen and frontier Li-ion anodes and cathodes
(3) Towards sustainable battery materials and processing
(4) Solid state batteries
This event has a joint program with this event on Solid State Batteries.
The conference covers the latest innovations and developments on applied research, materials, manufacturing and applications from around the world.
The programme is entirely curated by our in-house experts, striking a fine balance between industrial developments and applied research advancements, bringing together a world-class set of speakers from end users, material developers, manufacturers, start-ups, as well as renowned research centers and market analyst groups.
Our exceptional online events are also truly a unique networking opportunity.
All talks will be given live online but will also become available on-demand. The talks from previous events are all also accessible in your library with a single annual (virtual or hybrid) annual pass. You can see the past events here
2024 | Battery Materials: Next-Generation & Beyond Lithium Ion
2023 | Battery Materials: Next-Generation & Beyond Lithium Ion
2022 | Battery Materials: Next-Generation & Beyond Lithium Ion
* in the agenda means that the title is tentative awaiting final confirmation by the presenter

8inks
Paul Baade
Co-Founder

Multilayer curtain coating for lithium-ion batteries
Multilayer curtain coating as a manufacturing platform unlocks mass production of nextgen battery designs and materials. It allows cell manufacturers to tailor the layup and composition within electrodes and new functional layers can be integrated into the electrode coating step. Ultimately manufacturing costs can be reduced by 30% and battery performance can by improved by leaving production constraints behind. We enable more freedom of design for battery architectures that are optimized for its application with material flexibility.

AM Batteries
Yan Wang
Co-founder & President
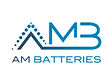
Dry Electrode Manufacturing Technology-An Enabler for Low Cost Batteries.
The global acceleration towards sustainable energy has driven the need for low-cost, de-carbonized battery manufacturing processes with differentiating product performances. AM Batteries unique dry electrode manufacturing technology aims to deliver on these critical challenges and elevate battery production and performance globally. Our liquid-free battery electrode manufacturing process entails 3 key steps: dry mixing, dry deposition, and mechanical compression. In this talk, we will explain our technology and highlight our achievements and manufacturing advantages over other electrode process options.

Altris
Dr. William Brant
Founder
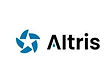
Empowering an Independent future: Industrializing Sodium-Ion Battery Technology with Altris
Discover how Altris is revolutionizing energy storage with its cutting-edge sodium-ion battery technology. This presentation will highlight the unique advantages of Altris’ patented cathode material and cell, its commitment to independent, sustainable and cost-efficient solutions, and its strategic approach to industrialization. Learn about Altris partnership model, and enabling commercial applications for an independent, greener, sodium-powered future. Gain insight into how Altris is meeting market demands, securing supply chains, and accelerating the transition to more sustainable energy storage solutions.

Benchmark Mineral Intelligence
Alex Albinski
Analyst
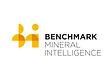
Anode Market Trends: The Rise of Synthetic Graphite?
Graphite is the most widely used anode active material in lithium-ion batteries. In 2024, anode producers increasingly switched to using more synthetic graphite relative to natural graphite, but what is driving this trend? Currently, natural and synthetic graphite account for 97% of total anode material supply but a host of alternative anode chemistries have emerged. What place do silicon engineered anode materials and other chemistries have in the current low-
price environment for graphite?

E-Lyte Innovations GmbH
Jonathan Bäthge

Enhancing battery safety in Sodium-Ion Batteries: Introduction of a novel electrolyte additive to suppress Sodium plating and improve lifespan
The electrolyte plays a crucial role in improving key performance indicators (KPIs) such as efficiency, cost, safety and lifespan of a Sodium-Ion Battery (SIB). By adjusting the electrolyte to prevent active material degradation and sodium plating on the anode, which represent currently occurring problems while cycling SIBs at high cut-off voltages, an enhancement in performance of SIBs can be achieved. Patent and literature analyses were conducted to identify relevant existing electrolyte components, providing a foundation for further electrolyte development and research on new additives. Therefore, new electrolyte additives were investigated to improve the safety and lifespan of SIBs.

Fraunhofer IFAM
Mario Kohl & Daniela Fenske
Printing
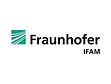
Printing technology as green alternative for thick layer Li Ion Battery electrode production
The need for more ecological and sustainable production technologies is a general demand for competitive battery manufacturing factories in the future. The energy consumption and carbon dioxide emissions are cost-driving factors and at the same time have high increasing social impacts. Solutions for making electrode production greener can be realized by avoiding or replacing toxic and harmful materials, e.g. by aqueous processing. Also less solvent requiring processes may lead to less extensive drying efforts. Screen-printing technology for electrode manufacturing combines both environmental benefits and enables for thick film electrode production with high areal capacities especially for high energy cells (up to 5…8 mAh/cm 2 ). In this work it will be shown that the solvent amount can be decreased by about 20% by processing of highly filled pastes. In addition, it is possible to enable a multilayer printing and therefore sequential and fast drying as well as near-net-shape printing leading to less waste upon production. The thickness and high areal capacity of the printed coatings lead to new design aspects of the electrode structure affecting full cell performance. Some insights will be given with regard to current-rate capability, useable capacity, porosity and efficiency of ion- exchange pathways. The screen-printing process is a scalable and established series production technology. The high accuracy, energy efficiency and low emissions are strongly in line with the goals of green production.

Georgia Institute of Technology
Matthew McDowell



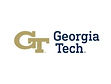
Interface Evolution in Solid-State Batteries
Solid-state batteries offer the promise of improved energy density and safety compared to lithium-ion batteries. However, the electro-chemo-mechanical evolution of materials at solid-solid electrochemical interfaces is different than at solid/liquid interfaces, and contact evolution in particular plays a critical role in determining the behavior of solid-state batteries. Using X-ray tomography, cryo-FIB, and finite-element modeling, we show that anode-free solid-state lithium metal batteries are intrinsically limited by current concentrations at the end of stripping due to localized lithium depletion. This causes accelerated short circuiting compared to lithium-excess cells. Based on these results, the beneficial influence of metallic interfacial layers on controlling lithium evolution and mitigating contact loss from localized lithium depletion will be discussed. We also show that alloy anodes in solid-state batteries can exhibit improved interfacial stability and enhanced cyclability compared to Li-ion batteries. A comprehensive study on 12 alloy materials shows that lithium trapping is a key limitation for most materials. Further characterization of alloy anodes reveals the dynamic nature of interfacial fracture during reaction processes. Taken together, these findings show the importance of controlling chemo-mechanics and interfaces in solid-state batteries for improved energy storage capabilities.

Innolith
Laurent Zinck
Chief Scientist
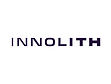
Next-Generation Li-Ion Batteries: High performance through 5V Stable and Highly Conductive Inorganic Electrolyte
Conventional lithium-ion batteries utilizing organic electrolyte suffer severely from several drawbacks associated to the poor electrochemical stability at higher potentials, high volatility and flammability of electrolyte components as well as poor performance at lower temperatures. To overcome these issues we developed a novel purely inorganic electrolyte consisting of sulfur dioxide as a solvent and a proprietary conductive salt. [1,2] This electrolyte formulation shows superior stability towards potentials of up to 5V together with exceptional ionic conductivity exceeding organic electrolyte by up to an order of magnitude. Throughout this presentation we will discuss in detail the basic properties and electrochemical performance of our SO2-based electrolytes in comparison to state of the art organic electrolyte. Extensive research efforts of the last few years have led to a unique electrolyte system, which is capable to withstand electrolyte decomposition beyond a potential of 5V. The larger potential window allows for higher average discharge potentials and significantly increased capacities. For NMC, for instance, a capacity increase of 20% is possible, which not only enhances cell energy but may also reduce cost due to more efficient cathode utilization. 21700 cells manufactured with our pilot-scale manufacturing equipment already achieved energy densities as high as 310 Whkg-1 with ordinary NMC/graphite cell chemistry. Recently, we have shown that our cells not only may be cycled for hundreds of cycles at 4.6 V but also demonstrate exceptional charge/discharge characteristics at temperatures as low as -40 °C. Our cells also passed all tests associated to UN38.3 which paves the way towards commercial applications. Our technology may be understood as a drop in solution for large scale battery cell manufacturing. Only small modifications with regard to electrolyte handling and filling have to be made by maintaining all other cell manufacturing processes such as electrode coating and cell assembly. This together with the prospect of proven compatibility with future electrode materials such as LMR, LNMO, LFMP, Silicon or lithium metal makes our technology highly adaptable and future proof.

Lawrence Livermore National Laboratory
Jianchao Ye
Scientist
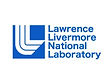
Laser Additive Manufacturing for Batteries
The development of cost-effective, high-performance battery manufacturing technologies is essential to support the U.S. electric vehicle, energy storage, and consumer electronics markets. Shifting from wet to dry processing for battery electrodes
promises to lower capital costs, energy usage, and environmental impact. This presentation explores our work on powder-based additive manufacturing techniques to produce cathode and solid electrolyte films without solvents. Using methods like selective laser melting and cold spray, these energy-efficient processes minimize material waste while achieving production speeds comparable to conventional slurry casting. I will discuss the additive manufacturing processes, the critical factors influencing film quality, and cell performance in comparison to traditional slurry-based methods. This work was performed under the auspices of the U.S. Department of Energy by Lawrence Livermore National Laboratory under Contract DE-AC52-07NA27344.

LionVolt
Sandeep Unnikrishnan
Co-founder & CTO

Enabling next generation Li metal batteries
World needs next generation batteries to address the energy density limitations of conventional lithium-ion batteries. Lithium metal anodes are considered to be holy grail for enabling next-gen batteries, but have challenges. This presentation will be about the challenges to be overcome to realise Li metal anode batteries, and how LionVolt is enabling it using its unique 3D electrodes to unlock the next generation of performance for a wide range of applications. The presentation will touch up the latest performance of LionVolt's 3D electrodes, including relative energy density and cost benefits. LionVolt’s 3D Li-metal anodes can feed into existing LiB cell production infrastructure as a drop-in solution and can be employed across lithium-ion chemistries and beyond, including sodium-ion and solid-state cells. LionVolt is completing the build of a new MWh 3D Li anode pilot line in the Netherlands to complement its cell manufacturing fab in the UK.

Lyten
Ratnakumar Bugga
Senior Fellow
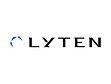
Lyten's Advancements in Lithium-Sulfur Batteries for Electric Vehicles
Lithium-sulfur batteries is an attractive battery technology, beyond the current Li-ion batteries with many advantages including high specific energy, low cost, improved safety, abundant raw materials that are not plagued by supply chain issues, and low carbon footprint. Yet, their implementation in industry has been challenging, despite impressive results in the academic environment, deterred mainly by the ‘polysulfide shuttle’ – a phenomenon attributed to the dissolution of polysulfides in electrolyte. To address this, Lyten has developed a unique family of 3D Graphene (3DG) materials from the cracking of hydrocarbons to produce hierarchical porous structure which can mitigate shuttle effects by physical entrapment of polysulfides. With its well-engineered pore structure with controlled nano/micro/meso pores providing high pore volume, Lyten 3D graphene can accommodate high proportions of sulfur and can improve retention of the polysulfide intermediates during cycling. Furthermore, its mechanically flexible framework counters the volume change of sulfur, and its high electronic conductivity overcomes the poor conductivity of sulfur and polysulfides. With the 3D graphene-sulfur cathode active material, Lyten has been fabricating high areal capacity cathodes using aqueous binders on industrial coaters. In parallel, Lyten is also developing other cell components, e.g., novel protected Li composite anodes including 3D architectures, advanced stable electrolytes that can function at electrolyte/sulfur ratios, and multi-functional separators that can block polysulfide crossover to the anode. Integrating these cell components and utilizing semi-automatic cell assembly lines, Lyten is making prototype cylindrical and pouch Li-S cells of 2-10 Ah, which show specific energies of 250-325 Wh/kg and cycle life of 250-300 cycles at C/3 and 100% depth of discharge (DOD), over 500 cycles at 50% DOD, >1000 cycles at 30% DOD and over 3000 cycles at 20% DOD in LEO (Low Earth Orbit) satellite tests. Safety tests performed on these cells, including internal short through nail penetration, external short, overcharge and over-discharge and crush showed impressive abuse tolerance without thermal runaway. In this paper, the highlights of our recent advances in the materials and component development, and the performance of cylindrical and pouch cells will be presented.

Nanode Battery Technologies
Jiankuan Li
Chief Scientist
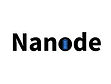
Beyond Lithium: Redesigning Sodium-Ion Architectures with Sn-Based Anodes via Streamlined Fabrication Techniques
Current lithium-/sodium-ion batteries (LIBs/SIBs) are unable to meet the rapidly-growing energy demand, and one of the major reasons originates from the limited specific capacity of carbonaceous anode materials. Therefore, manufacturers and researchers are shifting their focus towards materials with greater specific capacities. Among these materials, Sn has garnered increasing attention owing to its outstanding specific capacity in both LIBs (994 mAh/g) and SIBs (847 mAh/g) and excellent compatibility in both battery chemistries, showing great potential as one of the next-generation anode candidates.Nonetheless, its commercial use is significantly impeded by the capacity degradation during cycling. This degradation primarily results from the anode pulverization, triggered by the huge volume changes during charging and discharging. To address this problem, a novel nanostructured free-standing Sn-based ribbon is developed via a fast, one-step and solvent-free melt spinning process, and can be directly applied as anode, without the need of conventional electrode making and use of current collector. The anode pulverization issue has been significantly mitigated by the well-designed nanostructures and alloy phases, as well as other effective optimization strategies. As a result, the ribbon anode has been demonstrated in LIBs and SIBs and shown substantial improvements in specific capacity, cycle life, and rate capabilities.
In this talk, we will provide a brief overview of the melt spinning technique and some of our recent work in the development of ribbon anodes. We hope these findings can offer new insights into developing “next-generation” high-performance alloy-based anode materials.

Oak Ridge National Laboratory
Ethan Self
R&D Associate- Materials Electrochemist

Solution-Based Synthesis of Disordered Rocksalt (DRX) Li-ion Cathodes
Li-ion batteries containing conventional cathodes are unable to meet projected energy demands due to overreliance on critical resources—namely Co and Ni. Disordered rocksalt (DRX) materials represent a promising class of next-generation cathodes due to their high specific energy (>700 Wh/kg) and compatibility with earth-abundant transition metals (e.g., Mn and Ti). Despite these promising attributes, a major limitation of DRX cathodes is the lack of scalable synthesis platforms which enable fine tuning of the material’s structure and Performance. To address this issue, the present study reports the synthesis and characterization of Mn-based DRX oxyfluorides prepared through a scalable two-step route involving: (i) a solution-based combustion reaction to prepare a transition metal oxide precursor, followed by (ii) a high temperature reaction with lithiation/fluorination agents. Overall, the approach yields high purity DRX powders which can be prepared at lower temperatures and over shorter timeframes (e.g., 800 °C and 1 h) compared to conventional solid-state processes. Interestingly, these findings demonstrate that adding LiF to the oxide precursor is critical to facilitate DRX phase formation during the second heating step. These Mn-based DRX cathodes exhibit stable cycling performance with reversible capacities up to ~215 mAh/g in Li metal half cells. This presentation will discuss recent findings for DRX cathodes produced through this two-step reaction route. More specifically, effects of precursor selection and annealing profile on the reaction pathway and electrochemical performance will be highlighted. Overall, these results illustrate the merits and opportunities for scalable combustion reactions to produce Co/Ni-free DRX cathodes.

Orion Carbons
Dietmar Jansen
Chemist
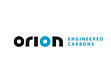
Engineered Carbon Blacks for Energy Storage Applications
Carbon Black is a critical component in lithium batteries, regardless of the specific technology employed, including Li-ion, solid-state, and post-Li. Despite the low ratio at which carbon black (CB) is added, it plays an indispensable role in the battery. To date, CB has not been a primary focus of LIB research. This perspective has shifted in light of the advancements in engineering that have reached a point where even inactive materials must be optimized to enhance key parameters in battery technology. This presentation will provide an overview of the underestimated, wide-ranging applications of CB, the crucial key attributes of CB for batteries, and their impact on the application field. This will also illustrate how the properties can be tailored to fulfill the different requirements of battery manufacturing steps. Examples of several CBs will be presented to demonstrate the possibilities of a customized production process.

P3 automotive GmbH
Behnoosh Bornamehr
Consultant
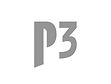
Next-gen battery strategies 2027+: Potentials and challenges for future battery designs and diversification in product portfolios to serve a large bandwidth of market applications
Revolutionizing Automotive Energy Storage: Investigating the integration of Cell-to-Pack (CTP) and Cell-to-Chassis (CTC) concepts for heightened energy densities and cost efficiency, coupled with a heightened emphasis on mixed cell chemistry concepts both on pack and cell level. Next-gen technology development: Navigating fluctuating raw material prices with Sodium-Ion Batteries (SIB) and Embracing Innovations in Anode Engineering and (Semi-)Solid-State technology for diverse applications including EV business and beyond. Diversification of cell manufacturer’s product portfolio: Increasing significance of market segmentation addressing the variety of needs for applications such as Bus, Truck, Off-Highway and ESS, and the standard EV business.

Pacific Northwest National Laboratory
Dongping Lu
Senior Scientist/Lead
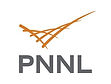
Cathode Development for High-Energy All-Solid-State Lithium-Sulfur Battery
The All Solid-State Lithium-Sulfur Battery (ASSLSB) holds great promise in delivering a safe, high energy
density, and low-cost battery technology suitable for vehicle electrification and grid energy storage. To
achieve a practically high specific energy in ASSLSBs (e.g., >400 Wh/Kg), it is essential to develop sulfur cathodes with high areal capacities (>6 mAh cm⁻²) to offset the relatively low working voltage of
elemental sulfur cathodes (1.9 V vs. Li). This necessitates the development of high mass-loading sulfur
cathodes by maximizing both the sulfur content in the electrode and the sulfur utilization rate to reduce
parasitic weights. An optimal electrode architecture featuring sufficiently connected sulfur/solid electrolyte/carbon triple-phase boundaries (TPBs) is crucial to enable rapid electron and Li-ion accessibility to the sulfur simultaneously. However, due to the non-flowable nature of these three solid components, it is challenging to maximize the contact among the solid phases, which requires new insights into materials design and processing technologies. Our research focuses on screening the cathode materials and their impact on the TPBs between electrochemically active sulfur, carbon, and the solid-state electrolyte (SSE) that comprise most composite cathodes in ASSLSB systems. We have developed optimal cathode materials and compatible SSEs to enhance TPB density, achieving an extremely high sulfur utilization rate in high mass-loading sulfur electrodes. The details of our study will be presented and discussed at the meeting.

SIONIC ENERGY
Karthik Ramaswami
CTO
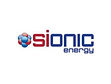
On the Road to Commercializing Graphite-free 100% Silicon Batteries
Congratulations, you have developed a great Silicon anode material! Mission accomplished? Not so fast! At best, you’re less than half-way to a product.
It is a long and winding, pot-hole filled road from having a good performing active material to a differentiated, practical battery that can be manufactured at scale and at a reasonable cost. This presentation will walk the audience through the key challenges encountered in developing
a superior, graphite-free Silicon battery delivering industry leading energy density of 370 Wh/Kg,
high power density and exceptional cycle life using commercially available materials and manufacturing processes. Did we mention the pouch cells need no external stack pressure?

Sakuu
Arwed Niestroj
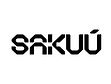
Reinventing manufacturing for better batteries
For decades the manufacturing process of batteries and its components has shown only incremental improvements to cost efficiency, energy efficiency and low yield rates. It requires a significant technology step to harvest new efficiencies in capex, opex, sustainability and new battery storage solutions. Advances in battery material science combined with experience from the semiconductor manufacturing industry now enable significant progress in electrode manufacturing, new battery concepts, solid state batteries and safer energy storage.
Several technology players have been attempting to establish and scale a dry electrode manufacturing process that overcomes the high energy demand, low reliability, low flexibility, high carbon footprint and use of toxic materials of today’s wet coating processes. The biggest advances though, are only possible by combining material preprocessing and new high speed additive manufacturing. Dry electrode printing reduces energy consumption and carbon footprint, eliminates all use of toxins and forever chemicals while delivering full scalability and high volume cost efficiencies. Dry printing of electrodes is the first step to printing complete batteries, in arbitrary shape and form, including SSB materials, eliminating metal foils and integrate inherent safety features – all at competitive high volume efficiency.

Solidion Technology, Inc.
Bor Jang
Chief Science Officer




Elastic Polymer-Encapsulated Micron-Scaled Si Particles: A Low-Cost Solution to High-Energy Lithium-ion Batteries

Stellantis
Luca Belforte



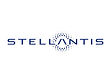
Sustainable materials and advanced characterization for the next generation batteries

Stratus Materials
Jay Whitacre
CEO/CTO
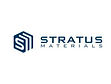
Stabilized Cobalt-Fee Lithium-Rich Cathode Materials; Using Process Innovation to Create a Safe and Practical Next Generation Battery Material.
This talk will cover exciting new results from Stratus Materials showing the performance of our next generation of cathode active materials. We will show how enhanced processing innovations result in materials approaching or exceeding 1000 Wh/kg at a materials level that have excellent performance in a range of use cases. Specifically, data showing that this material is stable in full cells for well over 1000 cycles will be disclosed. Other key metrics such as rate capability, fast charge capability, and manufacturability, and performance in thick-format electrodes will also be discussed. We will also explore the pack-level advantages of using this material using both safety and techno-economic assessments; pack-level energy densities that far exceed those of packs that use LFP and NMC cells are possible due to the energy densities and thermal performance attributes attained. Data from cells up to 20Ah in size will be shared.

UNIGRID Battery
Darren Tan
Chief Executive Officer

Advanced Na-Ion Batteries for clean Mobility & Storage
Advanced sodium ion batteries have already achieved energy densities competitive with lithium ion, however, despite promises of its improved safety properties, its safety metrics have not yet been clearly defined. This session will comprehensively describe the root causes of battery thermal runaway, compare the safety abuse data sets of various sodium ion battery chemistries. It will also cover recent trends in the sodium battery markets and how the sodium supply chain can alleviate today's critical material bottlenecks faced by the industry.

Washington University in St. Louis
Dr.Ruoxi Chen



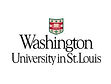
High-Capacity Half-Flow Li-02 Battery

Yale University
Liangbing Hu
Professor
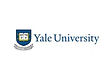
Cellulose Batteries
I will discuss a general design strategy for achieving one-dimensional (1D), high- performance polymer solid-state ion conductors through molecular channel engineering, which we demonstrate via Cu 2+ -coordination of cellulose nanofibrils. The cellulose nanofibrils by themselves are not ionic conductive; however, by opening the molecular channels between the cellulose chains through Cu 2+ coordination we are able to achieve a Li-ion conductivity. This improved conductivity is enabled by a unique Li + hopping mechanism that is decoupled from the polymer segmental motion. Also benefitted from such decoupling, the cellulose-based ion conductor demonstrates multiple advantages, including a high transference number (0.78 vs.0.2–0.5 in other polymers 2 ), low activation energy (0.19 eV), and a wide electrochemical stability window (4.5 V) that accommodate both Li metal anode and high-voltage cathodes. Furthermore, we demonstrate this 1D ion conductor not only as a thin, high-conductivity solid-state electrolyte but also as an effective ion-conducting additive for the solid cathode, providing continuous ion transport pathways with a low percolation threshold (Nature, Oct 22).